Key points
- Passive heating, or passive solar heating, means trapping heat from the sun inside your home and using thermal mass, heat flow and insulation effectively to store, distribute, and retain the heat.
- Passive heating can significantly reduce your energy bills. It is useful in nearly all climates, and especially useful in cool or cold climates.
- It is best to use passive heating design principles when building or buying a home, but some aspects of the principles can be used in home renovations.
- The main methods to achieve passive heating are to place living areas on the north side of your home, and include north-facing windows that let sunshine reach areas of thermal mass. You can also locate heaters near areas of thermal mass.
- Good insulation and appropriate glazing will help to ensure that the solar warmth stays inside your home.
- You can also stop cold air from entering your home by including airlock rooms, and sealing around doors, windows and your roof space.
Understanding passive heating
What is passive heating?
Passive heating uses free heating direct from the sun to dramatically reduce the cost of heating your home. Passive heating is most appropriate in mixed (temperate) or cold climate zones (Australian climate zones 4–8).
Effective passive heating has 5 main elements:
- Letting the sun in to your home - solar radiation travels through glass areas exposed to full sun. Window orientation, shading, frames and glazing type will all affect how well this process works.
- Storing the sun’s heat inside your home - trapped heat is absorbed and stored by materials with high thermal mass inside the home. This heat is released at night when the house starts to cool down.
- Ensuring good heat distribution - heat released from thermal mass is distributed to where it is needed through good design of air flow and convection. Direct re-radiation to areas of need is most effective, but heat can also be conducted through building materials and distributed by air movement. Floor plans should be designed to ensure that the most important rooms (usually living areas used during the day and evening) face north and receive the best winter solar access.
- Ensuring that heat is not lost - appropriate house shape and room layout will reduce heat loss. Heat loss occurs from all parts of the building, but mostly through the roof. In cool and cold climates, compact building shapes that minimise roof and external wall area are more efficient. Heat loss is further minimised with appropriate choice of windows and curtains or blinds, and well-insulated walls, ceilings and floors.
- Ensuring that cold air does not enter your home - infiltration of cold air is minimised with airlocks, draught sealing, airtight construction detailing and high-quality windows and doors.
Why is passive heating important?
Passive heating is the cheapest way to heat your home. It is:
- achievable and low cost when designed into a new home or addition
- appropriate for all climates where winter heating is required
- achievable using all types of Australian construction systems
- achievable when buying an existing house or apartment, by looking for good orientation and shading
- achievable when buying a home off the plan, by paying attention to correct orientation, making slight floor plan changes and choosing appropriate glazing
- possible to retrofit some aspects of passive heating design into an existing home.
Photo: © Tatjana Plitt Photography
Passive heating principles
Greenhouse principles
Passive heating design relies on greenhouse principles to trap solar radiation. Heat is gained when shortwave radiation from the sun passes through glass, where it is absorbed by building elements and furnishings and re-radiated as longwave radiation. Longwave radiation cannot pass back through glass as easily as shortwave radiation, so the temperature inside the room increases.
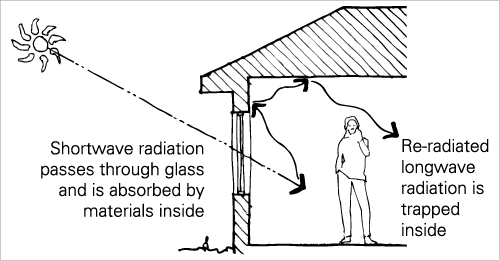
Thermal mass and thermal lag
Thermal mass is the ability of a material to store heat. When used correctly, thermal mass can significantly increase comfort and reduce energy use in your home.
Tip
High thermal mass inside the home (for example, brick walls or tile floors) can store heat from the sun during the day and release it when it is required, to offset heat loss to colder night-time temperatures or cloudy days. It effectively ‘evens out’ temperature variations between day and night.
This is because of a property known as thermal lag — the amount of time taken for a material to absorb and then release heat, or for heat to be conducted through the material.
Thermal lag times are influenced by:
- conductivity of the material
- difference in temperature (known as the temperature differential, ΔT or delta T) between each face of the material
- thickness of the material
- surface area of the material
- texture, colour and surface coatings (for example, dark, matt or textured surfaces absorb and re-radiate more energy than light, smooth, reflective surfaces)
- exposure of the material to air movement and air speed.
Source: Sustainable Energy Authority Victoria
Thermal mass is very useful for some climates, but can be a liability if used incorrectly. Thermal mass must be exposed to the home’s interior (so solar heat can flow easily into the material) and externally insulated (so the stored heat is not lost).
Rates of heat flow through materials are proportional to the temperature differential between each face of the material. External walls have much greater temperature differential than internal walls, which is why thermal mass must be insulated externally. The more extreme the climate, the greater the temperature differential and the more insulation required. Even 300mm-thick adobe and rammed earth walls need external insulation in cool and cold climates.
The useful thickness of thermal mass to be used in passive heating is the depth of material that can absorb and release heat during a day−night cycle. For most common building materials, this is 50−150mm (depending on their conductivity). Longer lag times are useful for lengthy cloudy periods, but must be matched by solar input (that is, if the material is needed to release heat over a long period, it will need to be able to first absorb that amount of heat.
Relative thermal lag of some common building materials
Material |
Thickness (mm) |
Time lag |
---|---|---|
Double brick |
220 |
6.2 hours |
Concrete |
250 |
6.9 hours |
Autoclaved aerated concrete (AAC) |
200 |
7.0 hours |
Adobe |
250 |
9.2 hours |
Rammed earth |
250 |
10.3 hours |
Compressed earth blocks |
250 |
10.5 hours |
Sandy loam |
1,000 |
30 days |
Source: Baggs et al. (2009)
In mild climates with low day–night temperature ranges, low-mass solutions with high insulation levels work well. Low-mass construction responds faster to internal heating and is more flexible as the climate warms. Flexible thermal mass options are also available.
In warmer temperate climates, external wall materials with a minimum thermal lag time of 10 to 12 hours can even out day–night temperature variations.
Extremely high thermal mass levels (for example, earth-covered housing) can even out high temperature variations between seasons. Summer temperatures warm the building in winter and winter temperatures cool it in summer. In these applications, lag times of 30 days are required.
However, the use of high-mass solutions may be less suitable in the future, as climate change increases summer temperatures and causes longer and more extreme heatwaves. In general, moderate mass or well-insulated lightweight construction is a more appropriate solution for the lifespan of housing built today.
Photo: Mike Cleaver, Clever Design
Heat flow
Heat is lost through building elements (for example, walls, floors, ceilings, windows) by conduction, particularly at night when the outside temperature is lower than the inside temperature.
Heat flow through any building element is directly proportional to the temperature difference on either side of that element. This is called the temperature differential (also referred to as delta T or ΔT). Temperature differential is like pressure in your garden hose. The greater the pressure, the more water flows through the hose. The greater the temperature differential, the greater the heat flow through the element.
The heat flow through different materials varies depending on their insulation properties (R value). Heat flow through windows is much higher because they usually have the lowest R value of any construction material.
Heat flow also varies depending on temperature stratification. Because hot air rises, air temperatures stratify in a home, with the hottest air in the highest areas. This means that the temperature differential to the outside will be largest in those highest areas, and you may lose more heat through those areas.
For example, if you are trying to keep your main living area at around 22°C on a cold −5°C Canberra night, temperature stratification might lead to 30°C at the highest point in the room and 18°C at the lowest. That means that 33% more heat may be flowing through higher-level building elements than lower ones because the temperature differential is 33% higher.
Heat loss and cold infiltration
Preventing heat loss is an essential component of efficient home design in any climate. It is even more critical in passive heating design because the main heat source is only available during the day. The building fabric must retain energy collected during the day for up to 16 hours and longer in cloudy weather.
Achieving passive heating
Passive heating requires careful application of the following passive design features:
- northerly orientation of daytime living areas
- thermal mass for storing heat
- appropriate areas of glass on northern walls
- passive shading of glass
- floor plan design to address heating needs
- insulation and draught sealing
- attention to air flow and infiltration.
Passive heating maximises winter heat gain, minimises winter heat loss, and concentrates heating where it is most needed.
Many of the features you need for passive heating (for example, orientation, thermal mass) should be designed into the home before it is built. But there are some changes you can make through renovation.
Orientation for passive heating
For best passive heating performance, daytime living areas should face north. Ideal orientation is true north, but orientations of up to 10° west of north and 20° east of north still allow good passive solar gain.
Where solar access is limited (for example, if the sun is blocked by nearby houses or other buildings, as is often the case in urban areas), you can still have an energy-efficient home through careful design. Homes on poorly orientated or narrow blocks with limited solar access can use alternative passive solutions to increase comfort and reduce heating costs.
Alternatively, you can use active solar heating systems that use roof-mounted, solar-exposed panels to collect heat and pump it to where it is needed to achieve energy efficiency on a difficult site. This solution can also be more easily adjusted to adapt to climate change, because the system can be switched off if it is not needed.
Thermal mass for passive heating
Locate thermal mass where it will be exposed to direct solar radiation or radiant heat sources (for example, near north-facing windows, or next to a heater).
Because thermal mass absorbs radiant heat as well as solar radiation, locating thermal mass walls between northern living areas and southern sleeping areas is a good idea. These will radiate daytime solar gains into sleeping areas at night through thermal lag, as well as providing a barrier to noise.
Air movement within the house heats or cools thermal mass. Locate the mass away from cold draught sources (for example, entries) and expose it to convective warm air movement in the house (for example, hallways to bedrooms).
Good thermal mass storage includes insulated or internal suspended slabs that are not earth-coupled, masonry walls, water-filled containers and newer phase-change materials.
Earth-coupled concrete slabs on ground are not an ideal storage medium for solar gain. They will absorb heat with very little temperature rise because they ‘wick’ heat away through earth-coupling. Their main role in passive design is to maintain overnight temperatures that can easily be topped up by solar gains or auxiliary heating the next day. In cold climates, insulate all slab edges and insulate underneath areas of the slab on the ground where the indoor surface is exposed to direct solar radiation. Consider using low thermal mass materials and high levels of insulation in south-facing rooms.
Photo: Amber Creative
Trombe walls
A trombe wall is a construction detail that collects heat in winter and can dump heat in summer, and can act as an additional temperature regulator.
Trombe walls have an outer skin of glass or clear polycarbonate, which allows heat to pass through to be absorbed by a high thermal mass internal heat bank. There is a small air gap between the clear outer skin and the thermal mass, which is usually a black-painted brick or mudbrick wall. The air gap allows direct solar gain to pass through the outer skin, to be absorbed and built up during the sunlit hours within the thermal mass, which then releases the gain slowly. The outer skin limits the heat passing back out, and thus most is retained inside the building. Trombe walls rely on the combined action of thermal lag of the mass and insulation from the air gap. Unwanted convection is controlled with openable vents at the top and bottom of the wall, which can dump accumulated heat from the interior overnight, if external temperatures are below those inside.
Normally installed on the northern façade, a trombe wall can be used in place of a solid wall, or in addition to windows. Trombe walls are normally limited in area to between 2 and 8 square metres, and need to be properly integrated into the cladding and glazing details to look attractive. Trombe walls must be effectively shaded to ensure the sun does not add heat in summer.
Photo: © AndyRasheed/eyefood
Windows and glazing for passive heating
Glazing is where most heat is lost and gained, because it transfers both radiant and conducted heat.
Tip
When deciding on glazing types and window sizes for solar heating, you will need to balance daytime heat gain against night-time heat loss. Large windows can facilitate solar gain, but they can also lose significant amounts of heat at night.
Avoid over-glazing — excessive areas of glass can be a large energy liability. In winter, there are 5 hours or fewer of solar heat gain but 19 or more hours of night-time heat loss. Look for products designed for cooler climates, such as products with low conductivity or U value (for example, double glazing) and high solar heat gain coefficients (SHGC).
Window frames also conduct heat. You can use timber, uPVC or thermally separated metal window frames in cooler climates (and hotter climates where air-conditioning is used) to prevent heat transfer.
Views are an important consideration and are often the cause of over-glazing or inappropriate orientation and shading. Plan carefully and explore advanced glazing and shading options to capitalise on views without decreasing energy efficiency.
Photo: Shutterstock
Glass-to-mass ratios
The ratio of solar-exposed glass to exposed thermal mass in a room is critical to energy efficiency. The ideal ratio varies significantly between climates.
Too much thermal mass for the available solar heat input creates a heat sink and increases the need for extra heating. Not enough thermal mass causes daytime overheating and rapid heat loss at night.
The amount of thermal mass used should be proportional to the day–night (diurnal) temperature range. Higher diurnal ranges (for example, in inland areas) require more mass; lower diurnal ranges (for example, in coastal areas) require less. As a rule of thumb, in climates where diurnal ranges are consistently less than 6–8°C, low thermal mass construction performs better. Consider the impacts of climate change when making decisions.
The area of north-facing glass with solar access should range between 15% (for temperate climates) and 25% (for cold climates) of the area of exposed thermal mass in a room.
You can analyse potential glass-to-mass ratios for your design. Nationwide House Energy Rating Scheme (NatHERS) software is most commonly used as a rating tool for council approval, as it can simulate the interaction of design variables for 69 different Australian climate subzones. Seek advice from an energy assessor accredited by the Australian Building Sustainability Association, Design Matters National or House Energy Raters Association who is skilled in using the software in non-rating mode.
Shading for passive heating
Passive heating should be used together with appropriate shading of windows to allow maximum winter solar gain and prevent summer overheating. This is most simply achieved with northerly orientation of appropriate areas of glass and well-designed eave overhangs.
Fixed horizontal shading, such as eaves, blocks summer sun (which is at a high angle) but lets in winter sun (which is at a lower angle). Fixed shading devices can regulate solar access to north-facing glass throughout the year, without requiring any effort. Correctly designed eaves are the simplest and least expensive shading method for northern elevations.
The ‘rule of thumb’ for calculating the width of north-facing eaves is shown in the following diagram. This rule applies to all latitudes south of and including 27.5° (Brisbane, Geraldton). For latitudes further north, the calculation will vary with the climate.
Permanently shaded glass at the top of the window is a significant source of heat loss. To avoid this, the distance between the top of glazing and underside of eaves or other horizontal projection should be 50% of overhang or 30% of window height, where possible.
Use adjustable shading to regulate solar access on other elevations. This is particularly important for variable spring and autumn conditions and allows more flexible responses to climate change.
Floor planning for passive heating
Plan carefully to ensure passive solar gain for the rooms that most need it.
Living areas and the kitchen are usually the most important locations for passive heating because they are used day and evening. In general, living areas should be placed along the north side of the home and bedrooms along the south or east side.
Bedrooms generally require less heating, because it is easy to get warm and stay warm in bed. Children’s bedrooms can be classified as living areas if considerable hours are spent there.
Utility and service areas such as bathrooms, laundries and garages are used for shorter periods, require smaller windows and generally require less heating. These areas are best located:
- to the west or south-west, to act as a buffer to hot afternoon sun and the cold westerly winds common to many regions
- to the east and south-east, except where this is the direction of cooling breezes.
Detached garages to the east and west can create protected north-facing courtyards, provide shade from low-angle summer sun and direct cooling breezes into living spaces. Consider adding a solar conservatory to maximise solar gains in cool climates. Ensure the heat that it traps can be distributed to thermal mass within the home during the day and that it can be sealed off from the rest of the house at night.
Compact floor plans minimise external wall and roof area, thereby reducing heat loss and construction cost. Determine a balance between minimising heat loss and achieving adequate daylighting and ventilation. Consider your specific regional heating and cooling needs and the site characteristics to determine an ideal building shape.
Tip
You will usually decide on a floor plan during the design and building phase, but you can also make changes to your existing home through renovation. Re-orientate as much of the living space as possible to the north side of the house. North-facing bedrooms can become living rooms, and south-facing living areas can become bedrooms. You can also add doors and walls to create zones with similar heating needs.
Source: © Beaumont Building Design
Airlocks
Airlocks are small closed areas at the entrance to a home that can be used to limit the entrance of cold air from outside and the loss of warm air from inside. In cool or cold climates, airlocks are a good idea at all external doors that are frequently used.
Main entry airlocks can include a small bench and storage spaces for coats, hats and boots. Airlocks at other entries can be double-purpose rooms: laundries, mud rooms and attached garages are excellent airlocks.
Allow sufficient space between doors so that it is easy to close the outer door before opening the inner door (or vice versa). Inadequate space often means inner doors are left open. Avoid using sliding doors in airlocks. They are often left open, are difficult to seal and cannot be closed with a hip or elbow when both hands are full.
Always design doors to blow closed if left open in strong winds, or consider using spring closers on external doors.
Locating heaters
Internal thermal mass walls provide an ideal location for heaters, especially radiant units such as wood heaters or hydronic heating panels. Thermal lag will transfer heat to adjoining spaces over extended periods
Locating heaters next to an outside wall can result in additional heat loss (unless they are well insulated), because increasing the temperature differential between inside and outside increases the rate of heat flow through the wall. Avoid locating heaters under windows.
Heaters create draughts when operating, so try to locate them where they can draw cooled air back through passageways rather than sitting areas.
Design for effective convection
Convection currents are created when warmer air from a heat source rises to the ceiling and air cooled by windows and external walls is drawn back along the floor to the heat source.
With careful design, convective air movement can be used to great benefit – convection can be used to distribute warm air and help to heat all areas of your home. But convection can be a major source of thermal discomfort if a home is poorly designed – convection can also cause draughts of cold air. Air movement creates a cooling effect on our bodies by increasing the evaporation of perspiration. Air movement of 0.5 metres per second (barely enough to move a sheet of paper) creates a cooling effect equivalent to a 3°C drop in temperature.
To make sure convection works effectively in your home, consider how air flows. Warm air flows will rise and flow along the ceiling to the highest point. You can visualise a helium-filled balloon riding the thermal currents – Where would it go? Where would it be trapped? Cool air flows are drawn by gravity and will fall towards the lower levels of your rooms.
Avoid convection draughts by designing floor plans and furnishing layouts so that cool air flowing from windows and external walls towards heaters or thermal mass sources is directed through traffic areas such as hallways and stairs, rather than living areas. Create draught-free nooks for sitting, dining and sleeping.
Use ceiling fans to circulate warm air evenly in rooms and push it down from the ceiling to living areas. For low ceilings, use fans with reversible blade direction to minimise draughts.
To improve heat distribution for single-storey homes:
- minimise cold convective air movement in winter with wall and ceiling insulation and glazing; the convection that occurs should provide passive heat distribution
- use controlled convection to warm rooms not directly exposed to heat sources
- if doors are often closed for privacy, include vents or openable panels (louvres or transom windows) over doors to facilitate air movement.
To improve heat distribution for multistorey homes:
- place most thermal mass and the main heating sources at lower levels
- use high insulation levels and low (or no) thermal mass at upper levels
- ensure upper levels can be closed off to stop heat escaping from living areas in winter and overheating in summer
- locate bedrooms upstairs in cold climates so they are warmed by rising air
- use stairs to direct cool air draughts back to heat sources, located away from sitting areas
- avoid open rails on stairwells, balconies and voids; they allow cool air to fall into spaces below
- use ceiling fans or heat shifters to push warm air back to lower levels
- minimise window areas at upper levels and use double glazing
- use close-fitting curtains with snug pelmet boxes, or insulating blinds
- maximise the openable area of upper level windows for summer ventilation, and avoid fixed glazing.
Insulation for passive heating
High insulation levels are essential in passive solar houses. Try to insulate above the minimum levels required by the current National Construction Code.
Consider insulation for all relevant housing elements:
- Ceilings and roof spaces account for 25–35% of winter heat loss. To prevent heat loss, place most of the insulation next to the ceiling because this is where the greatest temperature control is required.
- Floors account for 10–20% of winter heat loss. In cool climates, insulate the underside of suspended timber floors and suspended concrete slabs. Insulate the edges of ground slabs. Insulation is not required beneath earth-coupled concrete slabs; however, it may be desirable when groundwater is present Waffle pod construction helps to insulate under slabs.
- Walls account for 15–25% of winter heat loss. Insulation levels in walls are often limited by cavity or frame width. In cold climates, alternative wall construction systems that allow higher insulation levels are recommended.
In high-mass walls such as double brick, rammed earth and reverse brick veneer, thermal lag slows heat flow on a day−night basis. Insulation is still required for most high-mass walls; strawbale walls are an exception as they have a high insulation value. Internal walls and floors between heating and non-heating zones can be insulated to minimise heat loss and help to reduce noise.
- Thermal mass must be insulated to be effective. Slab-on-ground edges should be insulated in colder climates, or when in-slab heating or cooling is installed within the slab.
Sealing your home for passive heating
While no home should be completely sealed to air flow, reducing the amount of warm air escaping to the outside can improve energy efficiency.
Draughts
Air leakage accounts for 15–25% of winter heat loss in buildings (CSIRO 2015). Reduce air leakage in your home by:
- choosing high-quality windows and doors with airtight seals
- using airtight construction detailing, particularly at wall−ceiling and wall−floor junctions
- using tight-fitting floorboards and insulating the underside of timber floors
- avoiding downlights that penetrate ceiling insulation
- avoiding open fires, and fitting dampers to chimneys and flues or blocking them off if unused
- avoiding permanently ventilated skylights
- ducting exhaust fans and installing nonreturn baffles
- sealing gaps between the window and door frames and the wall before fitting architraves in new homes and additions
- improving the performance of existing windows and doors by using draught-proofing strips. Install these between the door and frame, at the door base and between the openable sash of the window and the frame.
- sealing air vents; use windows and doors for ventilation as required. However, this may not be advisable for homes with unflued gas heaters.
Source: Sustainable Energy Authority Victoria, updated in Energy Smart Housing Manual 2020
Roof space
Some heat always escapes through your insulation into the roof space. If the roof space is sealed, this escaping heat is trapped and can raise the temperature of the roof space by up to 17°C.
References and additional reading
- Australian Government, Department of Industry, Science, Energy and Resources, Households.
- Baggs S, Baggs D and Baggs J (2009). Australian earth-covered and green roof building, 3rd edn, Interactive Publications, Wynnum, Qld.
- Cole G (2011). Residential passive design for temperate climates. Acumen, EDG 66 GC, February, Australian Institute of Architects.
- CSIRO (2015). House Energy Efficiency Inspections Project [PDF].
- Department of the Environment, Water, Heritage and the Arts (2008). Energy use in the Australian residential sector 1986–2020, Canberra.
- Hollo N (2011). Warm house cool house: inspirational designs for low-energy housing, 2nd edn, Choice Books, New South Publishing, Sydney.
- Sustainability Victoria (2020). Energy smart housing manual.
- The Centre for Liveability Real Estate.
- Wrigley D (2012). Making your home sustainable: a guide to retrofitting, rev. edn Scribe Publications, Brunswick, Vic.
Learn more
- Check Australian climate zones and discover what is best for your region
- Read Passive cooling to find out how your home can be designed to work well in winter and summer
- Explore Thermal mass and Insulation for more ideas on how to trap heat in your home
Authors
Original author: Chris Reardon 2013
Edited: Kylie Evans, Biotext 2020